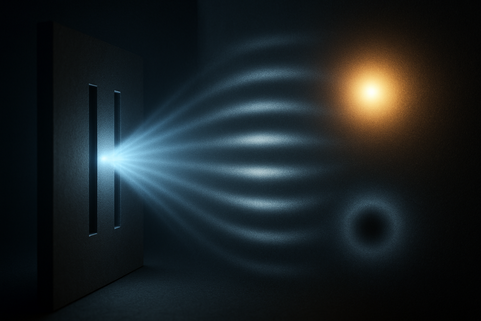
Bright and dark states of light: Rethinking a fundamental phenomenon
Thursday 01 May 2025Light. It illuminates our world, yet its fundamental nature has puzzled scientists for centuries. Is it a wave, spreading out like ripples on a pond? Or is it a particle, a tiny packet of energy called a photon? The answer, accepted for about a hundred years, is that it’s somehow both. This wave-particle duality is one of the cornerstones of quantum mechanics, often demonstrated by the famous double-slit experiment. But what if the classical wave interpretation of interference isn't the whole story? A recent development in quantum optics interference proposes a new way to look at this, suggesting that even classical interference patterns might have their roots in purely quantum particle states: bright and dark states of light.
Understanding light interference: The classic puzzle
Cast your mind back to 1801. Thomas Young performs an experiment that seems to settle the debate, at least for a while. He shines light through two narrow, parallel slits onto a screen behind them. Instead of two bright lines corresponding to the slits, he observes a pattern of alternating bright and dark bands, known as interference fringes. This looked exactly like how water waves interfere – where crests meet crests, the wave gets bigger (constructive interference, bright bands); where crests meet troughs, they cancel out (destructive interference, dark bands). Young’s experiment became textbook evidence for the wave nature of light.
Then came the quantum revolution. Einstein proposed that light energy comes in discrete packets – photons. Experiments later confirmed that even if you send photons through the slits one at a time, the interference pattern still builds up gradually on the screen. Each photon seems to ‘know’ about both slits, interfering with itself in a way that classical particles simply shouldn’t. This led to the concept of wave-particle duality: light behaves like a particle when detected, but travels and interferes like a wave. This remains a profoundly counterintuitive idea.
Waves, particles, and interference patterns
The classical wave explanation is neat: electromagnetic waves pass through both slits, spread out, and interfere. Where the electric fields of the waves add up, we get high intensity (bright fringes). Where they cancel each other out (destructive interference), the intensity is zero (dark fringes). In this classical view, if the electric field is zero in the dark regions, there should be no light energy there and no possibility of interaction with matter, like an atom placed in that region.
Quantum mechanics offers a different perspective. It describes the photon using a wave function, representing probabilities. The interference pattern reflects the probability of detecting a photon at different points on the screen. But quantum theory also throws a spanner in the classical works. It suggests that even where waves interfere destructively and the average electric field is zero, light particles might still interact with matter. There's a subtle but important difference between a zero average field and the quantum state itself. This discrepancy between classical wave predictions and potential quantum behaviour has been a point of theoretical discussion.
A new perspective: Bright and dark states of light
Now, a theoretical study by researchers Celso J. Villas-Boas, Gerhard Rempe, and colleagues, hailing from institutions including the Federal University of São Carlos, ETH Zurich, and the Max Planck Institute of Quantum Optics, offers a fresh photon interference theory. Published in Physical Review Letters, their work proposes that the familiar classical interference pattern can be entirely understood through the lens of quantum optics, specifically by describing light not just as waves, but in terms of collective quantum states of photons.
What are bright and dark states of light? The core idea is that photons arriving at the screen exist in specific entangled quantum states, which they term 'bright' and 'dark'. These concepts are analogous to 'bright' and 'dark' states of atoms described by Robert Dicke in the 1950s, but applied to optical modes containing photons.
- A bright state is a quantum state of light that can interact with a detector (in their model, a ground-state atom). If a photon arrives in a bright state at the atom's location, it has the potential to excite the atom.
- A dark state is a quantum state of light that cannot interact with that specific detector. This happens because the quantum mechanical amplitudes for excitation interfere destructively at the detector. Even if photons are physically present, their quantum state prevents them from coupling to, or 'being seen' by, the detector.
This new theory suggests that classical interference patterns directly correspond to these quantum states.
Interference patterns from particle states
How does this double slit experiment new theory explain the bands of light and shadow? It's remarkably direct:
- Bright Fringes (Maxima): These occur at positions on the screen where photons arrive in entangled bright states. These states readily couple to detectors (like the photographic plate, screen material, or a model atom detector), resulting in an observable signal – hence, a bright band.
- Dark Fringes (Minima): These occur where photons arrive in entangled dark states. Although photons reach these areas, their specific quantum configuration means they do not couple to the detector being used. They pass by unseen by that detector.
This leads to a startling question: Are there photons in dark interference regions? According to this dark states of light explained model, the answer is yes. Photons are predicted to be present everywhere the light wave reaches, including the nodes (dark fringes) of the interference pattern. The reason we don't see them there isn't because they are absent, but because they are in a quantum state that makes them effectively invisible to our detection method. As researcher Gerhard Rempe noted, this is "a highly counterintuitive picture". It challenges the classical idea that zero intensity means zero photons.
This framework provides a purely quantum mechanical explanation for a phenomenon long considered a hallmark of classical wave behaviour. It offers a classical interference quantum explanation based fundamentally on the particle nature of light (photons) and their specific quantum states, rather than relying on the classical wave picture of cancelling fields.
Solving long-standing paradoxes: The which-path mystery
One of the most baffling aspects of the double-slit experiment is the role of observation. If you try to detect which slit a photon goes through (gain 'which-path' information), the interference pattern disappears. The pattern reverts to two simple bands, as if the light were just classical particles. Why does finding out the path destroy the interference?
Traditional explanations often involve the idea that the measurement process inevitably disturbs the photon, for instance, by imparting a momentum kick that changes its trajectory and washes out the delicate interference. However, discussions, particularly in the late 1990s, highlighted that which-path observation could theoretically be done very gently, without a significant momentum kick. This raised an awkward question: if the particle isn't being physically jolted off course, how does the mere act of observation steer it away from the dark fringes and into the bright ones to destroy the pattern?
The bright and dark state theory offers a potential answer. How does which-path detection work in the new theory? It suggests the interaction involved in which-path detection fundamentally alters the quantum state of the photon. Specifically, the measurement interaction forces photons that would have arrived in a dark state (in a dark fringe) into a bright state. Crucially, this state change can happen without necessarily changing the photon's trajectory or giving it a momentum kick. The observation makes the previously undetectable photons detectable, effectively 'filling in' the dark fringes and thus destroying the interference pattern. The pattern vanishes not because the particle's path is crudely altered, but because its quantum state is changed by the measurement interaction itself.
The significance of the new theory
This new perspective on bright and dark states of light is significant because it attempts to bridge the gap between classical wave phenomena and quantum particle descriptions in a new way. It proposes a quantum origin for classical interference, rooted in the properties of entangled photon states and their interaction with detectors.
Researchers already knew that classical electromagnetism (Maxwell's equations) fails to capture many quantum optics phenomena. This new theory goes further, suggesting that Maxwell's equations might be viewed as a limiting case of a more fundamental quantum description. This deeper description emerges when two key factors are fully incorporated using quantum mechanics: 1. The detector monitoring the interference pattern and its quantum mechanical coupling to the light. 2. The description of interference as arising from entangled particle states (photons) rather than classical waves.
As Rempe puts it, "In some sense, we showed that Maxwell's equations are a limiting case of quantum mechanics." He views the description as meaningful because "it provides a quantum picture (with particles) of classical interference (with waves): maxima and minima result from entangled bright (that couple) and dark (that do not couple) particle states." He suggests this picture might resolve aspects of a debate involving scientific giants like Newton (who favoured particles), Maxwell (waves), and Einstein (who reintroduced particles via the photon).
Looking ahead: Future explorations
This work opens up intriguing avenues for future research. The current study focused specifically on light particles (photons) being observed by a simple model detector (a two-level atom in its ground state).
An obvious next step is to explore whether this concept of bright and dark states applies beyond photons. Could similar principles explain the interference patterns observed with material particles, like electrons or neutrons, which also exhibit wave-particle duality? Investigating this would require modelling different types of detectors suitable for matter particles, such as ionization devices or simply deposition on a surface.
Further theoretical development and potential experimental tests could help solidify or refine this interpretation. Does this particle-based view hold up under more complex scenarios? Can experiments be designed to probe the presence of photons in dark states more directly, perhaps using different types of quantum detectors?
While wave-particle duality is likely to remain a central concept in quantum mechanics, this new photon interference theory offers a different way to conceptualise how classical wave phenomena like interference emerge from the underlying quantum world. It reminds us that even foundational concepts in physics can be viewed in new ways, and the quantum realm likely still holds surprises in understanding the intricate behaviour of light and matter.
Bright and dark states of light: Rethinking a fundamental phenomenon
1. Physical Review Letters paper – DOI 10.1103/PhysRevLett.134.133603
2. arXiv pre-print (earlier version)
Comments
Post a comment…
A Serious Blog
News and views from around the world on lighting, eyecare and eye conditions.
Twitter: @seriousreaders
Facebook:facebook.com/seriousreaders
Recent posts
- The goal of a single-dose treatment for wet AMD
- A 7- to 8-fold higher risk of vision loss has been linked to this common drink
- Scientists pinpoint key gene in fight against dry AMD
- Bright and dark states of light: Rethinking a fundamental phenomenon
- Exploring photobiomodulation: A potential new direction for dry AMD research
Share your email address and we will keep you up to date as we learn more about light and offer you the best deals.